中国海洋湖沼学会主办。
文章信息
- 付倩倩, 李航霄, 吴海龙, 徐军田. 2018.
- FU Qian-Qian, LI Hang-Xiao, WU Hai-Long, XU Jun-Tian. 2018.
- 光强对缘管浒苔(Ulva linza)光合生理特性和短期温度效应的影响
- EFFECTS OF LIGHT INTENSITY ON PHOTOSYNTHETIC PHYSIOLOGY CHARACTERISTIC AND SHORT-TIME TEMPERATURE REACTION OF ULVA LINZA
- 海洋与湖沼, 49(5): 967-974
- Oceanologia et Limnologia Sinica, 49(5): 967-974.
- http://dx.doi.org/10.11693/hyhz20180400074
-
文章历史
- 收稿日期:2018-04-02
- 收修改稿日期:2018-05-16
大型海藻分布于近岸的潮间带和潮下带区域, 是海洋生态系统的重要组成部分, 占不到1%的海洋面积提供了约10%的海洋初级生产力(Smith, 1981; Dunck et al, 2015), 是海洋碳汇的重要组成部分(Raven, 2010)。许多大型海藻种类具有很高的经济价值, 是人类食品、工业原料及医药的来源之一(Pati et al, 2016; Wells et al, 2017)。大型海藻可以大量吸收海水中的氮、磷等营养元素, 在改善近岸海域的富营养化方面发挥着重要作用(Wu et al, 2015; Grote, 2016), 但同时导致了藻潮的暴发。浒苔作为一种典型的大型海藻, 具有广温、广盐的特征, 有较好的环境适应能力(Martins et al, 2002; 张寒野等, 2006), 并且具有较强的营养吸收能力, 在富营养情况下能够大量繁殖, 使其成为绿潮暴发种(Neori et al, 2004; Zertuche-González et al, 2009)。绿潮暴发对海洋中的生物危害很大, 它们会侵占底栖动物的栖息地, 导致其缺氧, 抑制其生长(Wallace et al, 2015)。同时绿潮暴发也影响人类的生活, 损害滨海旅游业, 破坏水产养殖产业(Zhang et al, 2010; Smetacek et al, 2013)。因此, 对以浒苔为代表的绿潮种进行暴发机制研究, 对于解释绿潮成因及灾害预测预警研究尤为重要。
但我们发现浒苔的暴发具有季节性和区域性, 这说明藻体的生长受光强和温度的影响, 光合作用是植物利用光能将二氧化碳转化为有机物, 是保证海藻生长和物质积累的生理基础(Collini et al, 2010)。光强是影响植物光合作用的重要的环境因素。当光照强度在一定的范围内, 光合作用随光强的增加而增加, 但光强超过一定的范围后, 光合作用就会降低, 出现光抑制(方同光等, 1964)。对于灾害藻, 占据优势的生理响应机制可以保证其有效应对环境胁迫, 保持正常生长。为应对高光强损伤, 浒苔可以通过上调LhcSR、PsbS和D1蛋白表达(Adir et al, 2003; Mou et al, 2013), 以及提高散热, 如叶黄素循环, 来增加非荧光化学淬灭(NPQ) (Heber et al, 2006)。光合作用还受溶解无机碳(DIC)的影响, 前人已对大型海藻如Gelidium canariensis、Gelidium arbuscula和Pterocladiella capillacea等进行了不同海藻对溶解性无机碳利用能力的研究(Badger et al, 1994)。
温度是影响植物光合作用的另一个重要的环境因子, 当瞬时温度增加, 净光合作用速率也会随之增加, 但当超过最适宜温度后, 净光合作用速率就会急剧下降。大型海藻的最适温度不是一个点而是一个温度范围, 同时不同的藻体都有自己的最佳温度区(Sage et al, 2007)。
虽然近年来研究表明占绝对优势绿潮种是浒苔(Ulva prolifera) (王宗灵等, 2018), 但缘管浒苔(Ulva linza)也是黄海暴发性绿潮的种类之一(Kim et al, 2011; Wang et al, 2015; Kang et al, 2016)。绿潮被广泛认可是来源于黄海南部浅滩筏式养殖区(Liu et al, 2009; Huo et al, 2013), 而缘管浒苔在这一区域占有一定的优势。本文从无机碳利用和对温度的短期效应方面研究不同光强水平对缘管浒苔的影响, 研究结果将为绿潮藻暴发机制的研究提供另一个视角。
1 材料与方法 1.1 实验材料缘管浒苔采自连云港高公岛海区(119.3°E, 34.5°N), 从中选取新鲜的、形态特征基本一致的藻体, 将其剪成2cm左右小段, 实验之前在培养环境下适应3天左右, 进行预培养培养介质为氮磷加富的灭菌自然海水(8μmol/L NaH2PO4和60μmol/L NaNO3)。
1.2 材料培养将藻体放在光照培养箱里培养, 并设置了三个不同水平的光强, 分别为90、200、400μE/(m2·s)。培养密度为0.06g/L, 通气培养, 光周期为L:D = 12:12, 培养温度为15℃, 每个处理设置三个重复, 每两天更换一次水。
1.3 实验方法 1.3.1 光合作用参数的测定光合指标测定采用YSI Model 5300 (维赛仪器, 美国), 通过低温恒温循环器(DHX-2005, 南京)来控制测定温度。不同水平的光强通过调节卤素灯的距离获得。
(1) 净光合作用速率和呼吸作用速率的测定
称量0.01g (FW)左右的藻体, 剪成大小均匀的小段, 在培养环境中适应1h左右, 以减少机械损伤造成的误差。测定反应介质为8mL的海水, 在完全黑暗的情况下测定其呼吸作用速率, 在培养光强下测定其净光合作用速率。
(2) 不同无机碳(DIC)浓度下净光合作用速率曲线(PC)的测定
通过改变反应槽中的无机碳的浓度, 从而测得不同DIC浓度下的净光合作用速率。首先制备无碳水, 同时配置NaHCO3母液。通过向反应槽中加入不同体积的NaHCO3母液从而得到不同的无机碳浓度, 测定不同DIC下藻体的净光合作用速率。相关参数根据米氏方程获得。

式中, V代表不同DIC浓度下藻体净光合作用速率; Vmax代表最大净光合作用速率; Km代表净光合作用速率达到Vmax一半时所需要的DIC浓度; S为底物(DIC)浓度。
(3) 短期不同温度下的净光合作用速率曲线(PT)的测定
温度对于生化反应的影响显著, 不同温度下, 藻体的净光合作用速率不同。通过设定低温恒温循环器的温度来改变反应槽的温度, 从而测定短期不同温度下缘管浒苔的净光合作用速率。通过Sharpe-Schoolfield公式进行拟合(Schaum et al, 2017)。

式中, b(T)是单个样品的代谢速率(μmol O2/(cell h); Ea是代谢过程的激发能; T是开氏温度(K); k是Botlzmann’s常数(8.62×10-5 ev/K); Th是酶半失活时的温度; Eh是当温度高于Th, 由温度诱导的使酶动力学失活的能量; Topt是藻体的最适生长温度; Tc=18℃, b(Tc)是从标准化到这个温度的代谢速率。
1.3.2 荧光参数测定荧光参数采用Dual-PAM-100 (Walz, 德国)测定。在测定之前需要对样品进行15min的暗处理, 测定其诱导曲线。光化光设置为培养光强, 得到NPQ和rETR, 将光化光设置为10个不同的光强(0、14、22、63、136、218、334、505、763、1182μE/(m2 s)。每个光强下进行短时适应(30s), 从而得到快速光响应曲线。
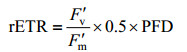
Fv′/Fm′指光系统Ⅱ (PSⅡ)的有效量子产量, 0.5表示50%的PAR分配到光系统Ⅱ中, PFD表示光合作用的光子通量密度。
用Jassby等(1976)的拟合公式进行拟合。

rETRmax表示最大相对电子传递速率; α表示在低光下的光能利用效率。
1.3.3 色素含量的测定取约0.02g (FW)的藻体, 用8mL的100%的甲醇溶解, 并将其暗置于4℃的冰箱中过夜, 测定在653和666nm波长下提取液的吸光度, 并按照Wellburn(1994)的公式计算叶绿素a和叶绿素b的含量。
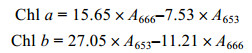
式中, A666和A653分别代表在波长666和653nm时的吸光值。
1.4 数据分析用Origin软件进行数据分析, 用单因素方差分析法(one-way ANOVA, Tukey)进行显著性分析, 设置显著水平为P<0.05。
2 结果 2.1 不同光强下的净光合作用速率与呼吸作用速率由图 1可知, 光强对净光合作用速率影响显著(P<0.05), 低光下净光合作用速率显著低于高、中光下, 与高光下净光合作用速率相比, 中光和低光下分别降低了6.47%和41.03%。光强对呼吸作用速率影响不显著(P>0.05)。
![]() |
图 1 不同光强下(90, 200, 400μE/(m2·s))缘管浒苔的净光合作用速率(A)和呼吸作用速率(B) Fig. 1 The net photosynthetic rate (A) and dark respiration rate (B) of U. linza under different light intensities 注:不同的小写字母代表处理间有显著性差异(P<0.05), 下同 |
由图 2可知, 光强对Fv/Fm值和NPQ值均影响显著(P<0.05)。高光下Fv/Fm值显著低于中、低光下(P<0.05), 而中、低光下Fv/Fm值无显著差异(P>0.05)。不同光强下NPQ值差异显著, NPQ值随光强的降低而显著降低(P<0.05), 中光和低光下的NPQ值相对于高光下的值分别下降了33.12%和81.34%。
![]() |
图 2 不同光强下缘管浒苔的最大光化学效率(Fv/Fm) (A)和非光化学猝灭(NPQ) (B) Fig. 2 The maximum photochemistry efficiency (Fv/Fm) (A) and non-photochemical quenching (NPQ) (B) of U. linza under different light intensities |
由图 3可知, 光强对Chl a和Chl b含量均影响显著(P<0.05)。低光下Chl a与Chl b的含量均显著高于高、中光下(P<0.05)。高光与中光下的Chl a的含量与低光下相比分别降低了53.39%和41.29%, Chl b的含量分别降低了77.70%和63.65%。
![]() |
图 3 不同光强下缘管浒苔的叶绿素a (A)和叶绿素b (B)的含量 Fig. 3 The Chl a (A) and Chl b (B) contents of U. linza under different light intensities |
中光下rETR最高, 光强对rETRmax和饱和光强(Ik)均影响显著(P<0.05) (图 4, 表 1)。不同光强下rETRmax差异显著(P<0.05), 高光和低光显著低于中光(P<0.05)。低光下Ik显著低于中、高光下(P<0.05), 但中、高光下Ik无显著差异(P>0.05), 藻体低光下的光能利用效率(α)在三个培养光强下均无显著性差异(P>0.05)。
![]() |
图 4 不同光强下缘管浒苔的光响应曲线 Fig. 4 The light curve of U. linza at different light intensities |
光强(μE/(m2 s)) | rETRmax | α | Ik |
90 | 116.36 ± 11.47a | 0.29 ± 0.02a | 410.14 ± 61.52a |
200 | 186.16 ± 19.20b | 0.29 ± 0.01a | 636.92 ± 67.63b |
400 | 161.20 ± 8.76c | 0.29 ± 0.03a | 570.28 ± 91.65b |
注: rETRmax表示最大相对电子传递速率; α表示在低光下的光能利用效率; Ik表示饱和光强。数据表现形式为平均值±方差, n=3, 不同的小写字母代表处理间有显著性差异(P<0.05) |
由图 5可以看出, 净光合作用速率随DIC浓度的增加而升高, 在达到饱和浓度后, 趋于稳定。净光合作用速率随生长光强的增加而增加。从表 2看出, 不同培养光强下的藻体的最大净光合作用速率Vmax差异显著, Vmax随培养光强的增加而显著增加(P<0.05), 低光培养下藻体的Km显著低于高、中光下(P<0.05), 而高、中光下Km差异不显著(P>0.05)。
![]() |
图 5 不同光强下的缘管浒苔在不同DIC浓度下的净光合作用速率(PC曲线) Fig. 5 The net photosynthetic rate of Ulva linza under different light intensities at different DIC concentrations (PC curves) |
光强(μE/(m2 s)) | Vmax | Km |
90 | 224.89 ± 4.48a | 0.049 ± 0.01a |
200 | 353.11 ± 2.19b | 0.131 ± 0.02b |
400 | 392.85 ± 24.54c | 0.169 ± 0.04b |
注: Vmax是最大净光合作用速率; Km是净光合作用速率为Vmax一半时的DIC浓度 |
由图 6看出, 不同培养光强下的缘管浒苔, 其净光合作用速率对温度变化的响应不同。低光培养下缘管浒苔的净光合作用速率的最适温度与中光培养下的相近, 但低于高光下。且低光培养下的缘管浒苔净光合作用速率明显低于高、中光培养下的藻体。由表 3可以看出, 诱导光合固碳酶失活一半时的温度(Th)和净光合作用速率的最适温度(Topt)受培养光强影响显著。高光下Th显著高于中、低光下(P<0.05)。而低光下Topt显著低于高、中光下(P<0.05)。
![]() |
图 6 不同光强下的缘管浒苔在不同的温度下的净光合作用速率(PT曲线) (温度表示为开尔文) Fig. 6 The net photosynthetic rate of Ulva linza under different light intensities at different temperatures (PT curves) (The temperature is Kelvin) |
光强 (μE/(m2 s)) |
90 | 200 | 400 |
Ea | 2.570 ± 2.630a | 1.153 ± 0.225a | 0.764 ± 0.079a |
b(Tc) | 10.299 ± 4.915a | 7.528 ± 0.606a | 6.636 ± 0.233a |
Eh | 7.113 ± 6.128a | 3.833 ± 0.545a | 2.8522 ± 0.116a |
Th | 279.747 ± 1.024a | 282.706 ± 1.526a | 286.002 ± 1.698b |
Topt | 278.536 ± 0.059a | 281.152 ± 1.198b | 283.533 ± 1.344b |
注: Ea是代谢过程的激发能; b(Tc)是从标准化到的18℃代谢速率; Tc=18℃; Th是酶半失活时的温度; Eh是当温度高于Th, 由温度诱导的使酶动力学失活的能量; Topt是藻体的最适温度(温度以开尔文表示)![]() |
藻体对光强的变化会显示出不同的反应。从结果中看出, 低光下, 藻体的净光合作用速率降低, 但色素含量增加, 使之能最大限度地吸收光能(Meyer et al, 2015); 高光与中光相比, 净光合作用速率无显著性差异, 但Fv/Fm显著降低, 说明缘管浒苔受到了高光胁迫, 光系统Ⅱ潜在的最大光能转换效率降低(汤文仲等, 2009), 这时植物本身的光保护机制就会发挥作用, 通过提高NPQ来降低高光对其的伤害(Xu et al, 2012)。
海水中的溶解无机碳虽然有约2mM, 但是绝大部分以HCO3-的形式存在, CO2所占比例不足1%。大型海藻Rubisco酶的Km值较高(40—70μmol/L), 对于只能依靠于CO2扩散的藻类通常在目前的海水碳浓度下是碳限制的(Ji et al, 2016)。然而, 在当前海洋无机碳浓度下, 很多大型海藻的光合作用能充分或者基本上达到饱和, 其生理原因是大多数藻类拥有CO2浓缩机制(CCM), 这种机制通常与高效的直接或者间接利用海水中的HCO3-库有关。我们研究发现, 低光培养下Km降低, 是由于光能不足, 藻体通过调节对无机碳的利用能力来提高CO2的利用效率, 是缘管浒苔适应低光环境一种策略。
光合作用对短期和长期温度变化反应的生理学研究, 了解海水的日常和季节变化对藻类的生产力和生存影响有重要意义(Sobrino et al, 2007; Figueroa et al, 2014)。在不同的温度下, 藻体通过一系列的机制来调整光合作用, 如调整色素含量和光合色素/光保护色素的比例(Falkowski et al, 1991)。低温下, 藻体需要更多的酶蛋白达到同样的催化活性, 特别是卡尔文循环(Gordillo et al, 2016), 虽然藻体短期暴露于高温下, 会显著增加光饱和的最大净光合作用速率(Pmax) (Zou et al, 2014), 但在对龙须菜的的研究中发现, 藻体只有在适宜温度范围内, 温度增加才会提高藻体的净光合作用速率(Liu et al, 2017)。本实验也说明了这个问题, 高于或低于最适温度时, 净光合作用速率都降低。当超过藻体的适宜温度, 温度越高, 藻体的净光合作用速率越低, 说明高温抑制光合作用中的Rubisco酶的活性, Rubisco酶的羧化能力降低, 同时高温引起CO2在水中的溶解度降低, 这也间接导致藻体净光合作用速率下降。当低于藻体的最适温度时, 低温改变Rubisco酶的动力学行为, 减少DIC的主动转运, 来维持CO2向Rubisco酶活力位点的供应。高光培养下, 海藻的光强诱导酶失活一半时的温度(Th)变大, 说明高光使海藻光合固碳酶对温度的耐受增强。低光培养下的海藻的净光合作用的最适温度显著低于高、中光培养, 说明低光下藻体的对高温比较敏感, 同时也表明高光下的藻体更能适应高温环境。
4 结论本研究表明, 低光培养导致海藻净光合作用速率的最适温度的下降, 但提高了其对无机碳的利用能力; 而高光培养导致光合固碳酶对温度的耐受性增强, 其光合固碳最适温度提高。这和黄海季节性变化是相吻合的, 在冬季光强很低, 同时温度也低, 这样缘管浒苔藻体的光合作用能力维持在一个相对低的水平, 在光强增加的春夏季, 温度进一步提升, 藻体在适应了高光强后, 对高温的敏感性也下降, 其光合作用维持在一个高的水平下, 从而使藻体快速生长, 在绿潮暴发的早期占据一定的优势, 但随着时间的推移, 缘管浒苔在绿潮种中所占的比例逐渐下降, 这在很大程度上是占绝对优势的浒苔种(Ulva prolifera)具有比缘管浒苔更有竞争性的营养盐吸收速率, 净光合作用效率和更强的漂浮能力(Luo et al, 2012; Huo et al, 2013; Wang et al, 2015; 王宗灵等, 2018)。
王宗灵, 傅明珠, 肖洁, 等, 2018. 黄海浒苔绿潮研究进展. 海洋学报, 40(2): 1–13 |
方同光, 张学明, 赵学武, 1964. 几种海藻的渗透生理与它们在潮间带分布的关系. 海洋与湖沼, 6(1): 85–96 |
汤文仲, 李信书, 黄海燕, 等, 2009. 不同光强和温度对长石莼(缘管浒苔)光合作用和叶绿素荧光参数的影响. 水产学报, 33(5): 762–769 |
张寒野, 吴望星, 宋丽珍, 等, 2006. 条浒苔海区试栽培及外界因子对藻体生长的影响. 中国水产科学, 13(5): 781–786 DOI:10.3321/j.issn:1005-8737.2006.05.014 |
Adir N, Zer H, Shochat S, et al, 2003. Photoinhibition-a historical perspective. Photosynthesis Research, 76(1-3): 343–370 |
Badger M R, Price G D, 1994. The role of carbonic anhydrase in photosynthesis. Annual Review of Plant Physiology and Plant Molecular Biology, 45(1): 369–392 DOI:10.1146/annurev.pp.45.060194.002101 |
Collini E, Wong C Y, Wilk K E, et al, 2010. Coherently wired light-harvesting in photosynthetic marine algae at ambient temperature. Nature, 463(7281): 644–647 DOI:10.1038/nature08811 |
Dunck B, Lima-Fernandes E, Cássio F, et al, 2015. Responses of primary production, leaf litter decomposition and associated communities to stream eutrophication. Environmental Pollution, 202: 32–40 DOI:10.1016/j.envpol.2015.03.014 |
Falkowski P G, LaRoche J, 1991. Acclimation to spectral irradiance in algae. Journal of Phycology, 27(1): 8–14 DOI:10.1111/j.0022-3646.1991.00008.x |
Figueroa F L, Barufi J B, Malta E J, et al, 2014. Short-term effects of increasing CO2, nitrate and temperature on three Mediterranean macroalgae:biochemical composition. Aquatic Biology, 22: 177–193 DOI:10.3354/ab00610 |
Gordillo F J L, Carmona R, Viñegla B, et al, 2016. Effects of simultaneous increase in temperature and ocean acidification on biochemical composition and photosynthetic performance of common macroalgae from Kongsfjorden (Svalbard). Polar Biology, 39(11): 1993–2007 DOI:10.1007/s00300-016-1897-y |
Grote B, 2016. Bioremediation of aquaculture wastewater:evaluating the prospects of the red alga Palmaria palmata (Rhodophyta) for nitrogen uptake. Journal of Applied Phycology, 28(5): 3075–3082 DOI:10.1007/s10811-016-0848-x |
Heber U, Bilger W, Shuvalov V A, 2006. Thermal energy dissipation in reaction centres and in the antenna of photosystem Ⅱ protects desiccated poikilohydric mosses against photo-oxidation. Journal of Experimental Botany, 57(12): 2993–3006 DOI:10.1093/jxb/erl058 |
Huo Y Z, Zhang J H, Chen L P, et al, 2013. Green algae blooms caused by Ulva prolifera in the southern Yellow Sea:Identification of the original bloom location and evaluation of biological processes occurring during the early northward floating period. Limnology and Oceanography, 58(6): 2206–2218 DOI:10.4319/lo.2013.58.6.2206 |
Jassby A D, Platt T, 1976. Mathematical formulation of the relationship between photosynthesis and light for phytoplankton. Limnology and Oceanography, 21(4): 540–547 DOI:10.4319/lo.1976.21.4.0540 |
Ji Y, Xu Z G, Zou D H, et al, 2016. Ecophysiological responses of marine macroalgae to climate change factors. Journal of Applied Phycology, 28(5): 2953–2967 DOI:10.1007/s10811-016-0840-5 |
Kang E J, Kim J H, Kim K, et al, 2016. Adaptations of a green tide forming Ulva linza (Ulvophyceae, Chlorophyta) to selected salinity and nutrients conditions mimicking representative environments in the Yellow Sea. Phycologia, 55(2): 210–218 DOI:10.2216/15-67.1 |
Kim J H, Kang E J, Park M G, et al, 2011. Effects of temperature and irradiance on photosynthesis and growth of a green-tide-forming species (Ulva linza) in the Yellow Sea. Journal of Applied Phycology, 23(3): 421–432 DOI:10.1007/s10811-010-9590-y |
Liu D Y, Keesing J K, Xing Q G, et al, 2009. World's largest macroalgal bloom caused by expansion of seaweed aquaculture in China. Marine Pollution Bulletin, 58(6): 888–895 DOI:10.1016/j.marpolbul.2009.01.013 |
Liu L, Zou D H, Jiang H, et al, 2017. Effects of increased CO2 and temperature on the growth and photosynthesis in the marine macroalga Gracilaria lemaneiformis from the coastal waters of South China. Journal of Applied Phycology, 30(2): 1271–1280 |
Luo M B, Liu F, Xu Z L, et al, 2012. Growth and nutrient uptake capacity of two co-occurring species, Ulva prolifera and Ulva linza. Aquatic Botany, 100: 18–24 DOI:10.1016/j.aquabot.2012.03.006 |
Martins I, Marques J C, 2002. A model for the growth of opportunistic macroalgae (Enteromorpha sp.) in tidal estuaries. Estuarine, Coastal and Shelf Science, 55(2): 247–257 DOI:10.1006/ecss.2001.0900 |
Meyer F W, Vogel N, Teichberg M, et al, 2015. The physiological response of two green calcifying algae from the Great Barrier Reef towards high dissolved inorganic and organic carbon (DIC and DOC) availability. PLoS One, 10(8): e0133596 DOI:10.1371/journal.pone.0133596 |
Mou S, Zhang X, Dong M, et al, 2013. Photoprotection in the green tidal alga Ulva prolifera:role of LHCSR and PsbS proteins in response to high light stress. Plant Biology, 15(6): 1033–1039 DOI:10.1111/plb.2013.15.issue-6 |
Neori A, Chopin T, Troell M, et al, 2004. Integrated aquaculture:rationale, evolution and state of the art emphasizing seaweed biofiltration in modern mariculture. Aquaculture, 231(1-4): 361–391 DOI:10.1016/j.aquaculture.2003.11.015 |
Pati M P, Sharma S D, Nayak L, et al, 2016. Uses of seaweed and its application to human welfare:A review. International Journal of Pharmacy and Pharmaceutical Sciences, 8(10): 12–20 DOI:10.22159/ijpps.2016v8i10.12740 |
Raven J A, 2010. Inorganic carbon acquisition by eukaryotic algae:four current questions. Photosynthesis Research, 106(1-2): 123–134 DOI:10.1007/s11120-010-9563-7 |
Sage R F, Kubien D S, 2007. The temperature response of C3 and C4 photosynthesis. Plant, Cell & Environment, 30(9): 1086–1106 |
Schaum C E, Barton S, Bestion E, et al, 2017. Adaptation of phytoplankton to a decade of experimental warming linked to increased photosynthesis. Nature Ecology & Evolution, 1(4): 0094 |
Smetacek V, Zingone A, 2013. Green and golden seaweed tides on the rise. Nature, 504(7478): 84–88 DOI:10.1038/nature12860 |
Smith S V, 1981. Marine macrophytes as a global carbon sink. Science, 211(4484): 838–840 DOI:10.1126/science.211.4484.838 |
Sobrino C, Neale P J, 2007. Short-term and long-term effects of temperature on photosynthesis in the diatom Thalassiosira pseudonana under UVR exposures. Journal of Phycology, 43(3): 426–436 DOI:10.1111/jpy.2007.43.issue-3 |
Wallace R B, Gobler C J, 2015. Factors controlling blooms of microalgae and macroalgae (Ulva rigida) in a eutrophic, urban estuary:Jamaica Bay, NY, USA. Estuaries and Coasts, 38(2): 519–533 DOI:10.1007/s12237-014-9818-1 |
Wang Z L, Xiao J, Fan S L, et al, 2015. Who made the world's largest green tide in China?-an integrated study on the initiation and early development of the green tide in Yellow Sea. Limnology and Oceanography, 60(4): 1105–1117 DOI:10.1002/lno.10083 |
Wellburn A R, 1994. The spectral determination of chlorophylls a and b, as well as total carotenoids, using various solvents with spectrophotometers of different resolution. Journal of Plant Physiology, 144(3): 307–313 DOI:10.1016/S0176-1617(11)81192-2 |
Wells M L, Potin P, Craigie J S, et al, 2017. Algae as nutritional and functional food sources:revisiting our understanding. Journal of Applied Phycology, 29(2): 949–982 DOI:10.1007/s10811-016-0974-5 |
Wu H L, Huo Y Z, Hu M, et al, 2015. Eutrophication assessment and bioremediation strategy using seaweeds co-cultured with aquatic animals in an enclosed bay in China. Marine Pollution Bulletin, 95(1): 342–349 DOI:10.1016/j.marpolbul.2015.03.016 |
Xu J T, Gao K S, 2012. Future CO2-induced ocean acidification mediates the physiological performance of a green tide alga. Plant Physiology, 160(4): 1762–1769 DOI:10.1104/pp.112.206961 |
Zertuche-González J A, Camacho-Ibar V F, Pacheco-Ruíz I, et al, 2009. The role of Ulva spp. as a temporary nutrient sink in a coastal lagoon with oyster cultivation and upwelling influence. Journal of Applied Phycology, 21(6): 729–736 DOI:10.1007/s10811-009-9408-y |
Zhang T T, Zheng C Y, Hu W, et al, 2010. The allelopathy and allelopathic mechanism of phenolic acids on toxic Microcystis aeruginosa. Journal of Applied Phycology, 22(1): 71–77 DOI:10.1007/s10811-009-9429-6 |
Zou D H, Gao K S, 2014. Temperature response of photosynthetic light-and carbon-use characteristics in the red seaweed Gracilariopsis lemaneiformis (Gracilariales, Rhodophyta). Journal of Phycology, 50(2): 366–375 DOI:10.1111/jpy.12171 |