中国海洋湖沼学会主办。
文章信息
- 张昊宇, 谭志军, 郑关超, 吕颂辉, 杨越聪, 吴海燕. 2023.
- ZHANG Hao-Yu, TAN Zhi-Jun, ZHENG Guan-Chao, LYU Song-Hui, YANG Yue-Cong, WU Hai-Yan. 2023.
- 13C示踪技术在利玛原甲藻中腹泻性贝毒合成研究的应用
- APPLICATION OF 13C TRACING TECHNOLOGY IN THE SYNTHETIC PATHWAY OF DIARRHEAL SHELLFISH TOXIN IN PROROCENTRUM LIMA
- 海洋与湖沼, 54(2): 457-464
- Oceanologia et Limnologia Sinica, 54(2): 457-464.
- http://dx.doi.org/10.11693/hyhz20220600169
文章历史
-
收稿日期:2022-06-27
收修改稿日期:2022-09-02
2. 农业农村部水产品质量安全检测与评价重点实验室 中国水产科学研究院黄海水产研究所 山东青岛 266071;
3. 青岛海洋科学与技术试点国家实验室 山东青岛 266071;
4. 暨南大学赤潮与海洋生物学研究中心 广东广州 510632
2. Key Laboratory of Testing and Evaluation for Aquatic Product Safety and Quality, Ministry of Agriculture and Rural Affairs, P. R. China, Yellow Sea Fisheries Research Institute, Chinese Academy of Fishery Sciences, Qingdao 266071, China;
3. Pilot National Laboratory for Marine Science and Technology (Qingdao), Qingdao 266071, China;
4. Research Center for Harmful Algae and Marine Biology, Jinan University, Guangzhou 510632, China
利玛原甲藻(Prorocentrum lima)是在海洋广泛分布的有害赤潮藻类, 是腹泻性贝毒(diarrhetic shellfish toxins, DSTs)的主要产生藻之一。DSTs属聚酮类毒素, 主要包括大田软海绵酸毒素(okadaic acid, OA)、鳍藻毒素1-3 (dinophysis toxins, DTX1-3)及其衍生物如酰化衍生物、7-O-酰基酯硫酸酯衍生物(sulfated dister)和乙二醇酯衍生物(diol esters), 如C8: 2OA、C9: 2OA、C10: 2OA、OA二醇酯和35S DTX1(Wu et al, 2020)。通常贝类摄食含有DSTs的藻类使其在贝类中蓄积, 被人类食用时可能导致腹泻性贝类中毒, 因此受到严格管控(Kilcoyne et al, 2020), 被两大国际有害藻华研究计划GEOHAB和GlobalHAB纳入重点研究对象。近年来研究对P. lima种群多样性(Nishimura et al, 2020)、产毒轮廓及其质量安全风险有了更为清晰的了解。现有研究表明所有P. lima均产OA, 其中部分藻株可产不同比例的DTX1 (Moreira-González et al, 2022)。同时P. lima是我国近海四大海域广泛分布的底栖有毒赤潮藻(Lim et al, 2019; Moreira-González et al, 2019; Nishimura, 2020; Gu et al, 2022), 已成为国家和社会共同关注的生态灾害和重大环境问题。
P. lima毒素的合成与营养盐的浓度和形态息息相关(杨维东等, 2008; 徐杏等, 2012; Lee et al, 2016; 曾玲等, 2017; David et al, 2018; 古松, 2018), 氮和磷盐限制均可促进P. lima中OA等毒素的生成。其中氮盐的主要形式包括NO3-、NO2-、NH4+ 等可溶性无机氮及尿素、可溶性游离氨基酸、核酸和腐殖质等可溶性有机氮(Bronk, 2002)。最近的研究发现, 许多浮游植物(特别是甲藻)偏向利用有机氮(Mulholland et al, 2004; Taylor et al, 2006)。尤其是在夏季可溶性无机氮浓度较低时, 高浓度的可溶性有机氮往往促进某些赤潮物种的繁殖(Flynn et al, 1986), 这可能与赤潮藻类对有机氮的利用有关(Berg et al, 1997)。氨基酸作为可溶性有机氮库中重要的组分, 广泛存在于天然水体、沉积物和大气中, 湖水中28%~46%的可溶性有机氮是氨基酸类有机氮(Yao et al, 2012)。研究表明不同的氨基酸之间也可以通过转氨基作用而互相转化, 氨基酸的分解代谢产生乙酰辅酶A和丙酮酸, 它们可以作为产毒藻毒素合成的起始物(Lee et al, 2016)。甘氨酸转移到线粒体后, 在甘氨酸脱羧酶和丝氨酸羟甲基转移酶的作用下, 可以将氮代谢与光呼吸相联系起来(Eckardt, 2005), 并且光合作用中的转运蛋白ABC家族直接参与了DSTs的转运(Hou et al, 2018; Gu et al, 2019)。在这一假设的前提下, Souto等(2001)的氨基酸添加实验得到了约两倍的毒素生成量。由此可见, 氨基酸可能是潜在的DSTs合成供体, 为DSTs合成路径的研究提供了新方法和思路。
稳定同位素示踪技术已被广泛应用于藻类毒素合成途径的研究。Anttila等(2016)使用13C-蛋氨酸培养亚历山大藻(Alexandrium)解析去甲基螺内酯C合成过程。Yamazaki等(2012)使用18O-醋酸酯解析了梯形聚醚——虾夷扇贝毒素(YTX)中的氧原子来源。高分辨液相色谱-质谱联用技术在稳定同位素标记方面具有很大的优势(Heuillet et al, 2018; Sun et al, 2020), 并且OA及其酯化态鉴定技术也有了最新进展(Wu et al, 2020)。本研究选取两株分布地域和产毒特征有较大差异藻株2XS株及SHG株, 应用13C稳定同位素示踪技术和高分辨质谱技术联合手段, 准确分析了甘氨酸培养下生长和毒素代谢谱变化, 为探究P. lima产毒代谢通路提供了新思路。
1 材料与方法 1.1 仪器与试剂DSP标准品: OA [C44H68O13, (8.4±0.4) μg/mL, m/z 805.473 3]; DTX-1 [C45H70O13, (8.5±0.7)μg/mL, m/z 819.488 9], 均购自加拿大国家研究理事会海洋生物科学研究所(CRM)。
化学试剂: 甘氨酸、2-13C-甘氨酸(99%纯度, ALDRICH公司)甲醇、乙腈和丙酮(HPLC级, 美国Merck公司); 甲酸铵(HPLC级, 美国Sigma Aldrich公司); 盐酸、氢氧化钠(优级纯, 国药); 吡啶(色谱纯, Duksan Pure Chemicals); 冰醋酸(分析纯, 天津市科密欧化学试剂有限公司)其他未作特殊说明的试剂均为分析纯, 实验用水为超纯水(18.2 MΩ.cm)。
1.2 藻种与培养基配制P. lima分别为2018年分离自渤海的SHG株(40°0′36′′N, 119°54′36′′E)和2015年分离自南海的2XS株(19°37′57′′N, 110°58′32′′E), 由暨南大学赤潮与海洋生物学研究中心提供。培养所用的海水取自青岛汇泉湾天然海水, 以0.45 μm混合纤维滤膜过滤后, 煮沸灭菌, 并转至高压灭菌的锥形瓶内, 接入f/2-Si培养基进行培养, 温度为25 ℃, 光照强度为125 μmol/(m2·s), 盐度28~32, 光暗周期为12 h︰12 h。分别以甘氨酸和2-13C-甘氨酸作为氮源对f/2中的氮源进行替换(882 mmol/L)(其余成分不变)。实验设立对照组(f/2), 甘氨酸培养组(gly)和2-13C-甘氨酸标记组(13C-gly)。
1.3 藻细胞培养与显微镜观察以10 000 cells/mL的密度接种于细胞培养瓶中接种, 每瓶100 mL, 每组3个平行。培养至21 d, 低功率超声(37 kHz) 30 s使其与培养瓶分离后摇匀使藻液充分混匀后(全部藻细胞悬浮)移取10 mL, 8 000 r/min离心10 min, 去上清液待测。取5 mL藻液于离心管中, 加100 μL鲁格试剂固定, 在TE100倒置显微镜(Nikon E100)下用浮游植物计数框计数, 记录细胞密度。
1.4 实验方法 1.4.1 样品前处理藻细胞加入5 mL甲醇, 低温超声破碎20 min (30%功率、5 s间隔), 用甲醇定容至10 mL, 过0.22 μm滤膜待测游离态DSTs; DSTs总量分析: 移取1 mL上述游离态DSTs提取液于螺纹口样品瓶中, 加入2.5 mol/L的NaOH溶液125 μL, 涡旋混合0.5 min, 密封于76 ℃下温育50 min。冷却至室温后, 加入2.5 mol/L的盐酸125 μL, 涡旋混合0.5 min, 过0.22 μm滤膜, 待测。
1.4.2 色谱-质谱条件Kinetex-C8柱(150 mm× 2.10 mm), 2.60 μm; 柱温: 40.0 ℃; 流速: 0.35 mL/min; 进样量: 10 μL; 流动相: A为水(含有2 mmol/L甲酸氨和50 mmol/L甲酸), B为95%乙腈水溶液(含2 mmol/L甲酸氨和50 mmol/L甲酸); 流动相梯度洗脱程序为0.00~0.50 min: 80% A; 5.00~13.00 min: 10% A; 13.10~15.00 min: 80% A。Q-Exactive液相色谱质谱联用系统(美国Thermo Fisher Scientific公司); 质谱条件: 加热电喷雾离子源(HESI), 扫描模式: 质量范围: m/z 600~1500; 喷雾电压3 500 V (正离子模式); 鞘气温度300 ℃; 一级全扫描(Full MS)分辨率为: 70 000 FWHM; 辅助气10 arb; 毛细管温度320 ℃; 数据依赖二级子离子扫描(dd-MS2, 分辨率为17 500 FWHM, C-trap最大容量(AGC target): 5×105, C-trap最大注入时间: 50 ms; 分步碰撞能量: 10/30 eV和20/40 eV。
1.5 数据分析实验数据采用origin软件进行数据处理。采用多因素方差分析方法和F检验分析比较各环境因子不同条件对两株藻生长和产毒影响的显著性, 按概率(P < 0.05)设置显著性检验阈值。
单细胞产毒量计算公式:
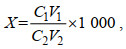
式中, X为最终样品藻细胞的毒素浓度, 单位: pg/cells; C1为测定样品藻细胞的毒素浓度, 单位: ng/mL; V1为样品提取剂体积, 单位: mL; C2为样品藻细胞密度, 单位: cells/mL; V2为样品藻液体积, 单位: mL。
同位素化合物的定量参考Cho等(2019)的研究并加以优化, 总结为公式如下:
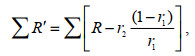
式中, r1为所求物质对照组12C离子质谱峰面积占所有同位素异构体质谱峰面积的比例; r2为所求物质13C标记后12C离子占所有同位素异构体峰面积的比例。∑表示对应成分的质谱峰面积。∑R′为修正后的质谱峰面积; ∑r1及∑r2表示对应比例所占的质谱峰面积; R=1, ∑R表示总质谱峰面积。
同位素标记相对丰度比值计算公式:
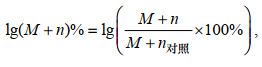
式中, M+n为该离子质谱峰面积; (M+n)%为该离子质谱峰面积所占同位素异构体质谱峰面积总和的比例; n=0~3。
2 结果与讨论 2.1 甘氨酸对藻细胞生长和产毒影响分析两株藻在f/2和gly培养基的生长情况及总产毒如图 1所示。不同藻株甘氨酸处理组与对照组生长无显著性差异(图 1a)(P>0.05), 而甘氨酸处理组产毒总量均显著提升(图 1b, 1c)(P < 0.05)。其中, SHG株的OA和DTX-1单细胞产毒总量分别达到7.88和4.35 pg/cell, 提升分别为16.9%和37.1%。2XS株仅产OA及其酯化态毒素, 其单细胞产毒量上升更为显著, 达到5.70 pg/cell提升了48.5%。
![]() |
图 1 不同处理组SHG株和2XS株的细胞密度、产毒和酯化态组成 Fig. 1 Growth, toxin production and esterification composition of SHG strain and 2XS strain in different treatment groups 注: a: 藻细胞密度; b: 总OA含量; c: 总DTX1含量; d: OA酯化态分布; e: DTX1酯化态分布; f/2: 对照组; gly: 甘氨酸培养组 |
OA和DTX1在ESI+模式下形成的分子离子峰包括[M+H]+、[M+NH4]+、[M+Na]+和[M+K]+峰, 其中[M+NH4]+峰强度最大。通过比较质量-电荷比(m/z)和保留时间(TR)来鉴定DSTs的毒素成分, 对其[M+NH4]+峰进行质谱分析, 如图 2所示两种藻类f/2组、gly组离子化模式一致且具有相同的同位素峰。二级质谱中OA(m/z 822.500 7, TR=5.40)主要的特征峰为m/z 223和267, DTX1 (m/z 836.516 2, TR=5.91)主要的特征峰为m/z 267, 此外两者还含有各自的脱水峰簇([M−nH2O+H]+)。采用上述定性判定依据及参考文献(Wu et al, 2020)的m/z值及RT值在两株藻中共分离鉴定16种不同的DSTs毒素种类(表 1)。将其响应强度与藻细胞密度相比, 得到相对响应响度(图 1d, 1e)。两株藻均含高比例酯化态成分, 2XS株和SHG株分别为88.1%、98.8%, 其种类及相对含量分布如图 1d、1e所示。SHG株含量较高的OA酯化态成分为C 9︰2 OA 1、C 9︰2 OA 2、C 10︰2 OA, 甘氨酸处理后产毒量分别提高39.8%、40.9%和29.5%。SHG株独有的C 9︰2 DTX1 1、C 9︰2 DTX1 2和C 10︰2 1成分, 分别上升了50.4%、15.5%和47.0%。2XS株主要酯化态毒素为C 9︰2 OA 1、C 9︰2 OA 2分别提高30.9%和50.1%。
![]() |
图 2 不同处理组OA和DTX1同位素分布及二级质谱图 Fig. 2 Isotopic distribution and MS/MS spectra of OA and DTX1 in different treatment groups 注: f/2: 对照组; gly: 甘氨酸培养组 |
化合物 | 分子式 | 保留时间/min | 预测分子量[M+NH4]+ /Da | 实测分子量[M+NH4]+ /Da | 质量准确度×10−6 |
OA | C44H68O13 | 5.4 | 822.499 8 | 822.500 7 | 0.48 |
OA甲酯 | C45H70O13 | 5.74 | 836.515 5 | 836.516 2 | 0.07 |
OA未知化合物 | C54H86O16 | 5.72 | 1 006.609 8 | 1 006.610 8 | 1.99 |
C8︰2 OA | C52H80O14 | 5.86 | 946.588 6 | 946.591 5 | 1.48 |
C9︰2 OA 1 | C53H82O14 | 5.91 | 960.604 3 | 960.607 4 | 4.88 |
C9︰2 OA 2 | C53H82O14 | 6.01 | 960.604 3 | 960.605 7 | 4.33 |
C9︰3 OA | C53H80O14 | 5.88 | 958.588 6 | 958.589 9 | 2.31 |
C10︰2 OA | C54H84O14 | 5.91 | 974.619 9 | 974.621 4 | 1.43 |
C11︰2 OA | C55H86O14 | 6.01 | 988.635 4 | 988.633 6 | 1.81 |
C11︰3 OA | C55H84O14 | 6.2 | 986.619 9 | 986.622 1 | 4.76 |
DTX1 | C45H70O13 | 5.91 | 836.515 5 | 836.516 2 | 0.07 |
DTX1甲酯 | C46H72O13 | 6.27 | 850.531 1 | 850.532 7 | 0.16 |
C8︰2 DTX1 | C54H84O14 | 6.26 | 960.604 3 | 960.605 8 | 4.33 |
C9︰2 DTX1 1 | C54H84O14 | 6.01 | 974.619 9 | 974.621 8 | 1.43 |
C9︰2 DTX1 2 | C54H84O14 | 6.34 | 974.619 9 | 974.621 8 | 1.43 |
C10︰2 DTX1 | C55H86O14 | 6.27 | 988.635 4 | 988.636 7 | 0.13 |
研究发现影响产毒因素主要有藻株生理特性(Nagahama et al, 2011; Zhang et al, 2015)和环境(David et al, 2018)。在甘氨酸作用下, P. lima单细胞产毒量上升, 且其细胞密度并未受到影响, 这意味着甘氨酸可能对P. lima产毒合成途径有着促进作用或是其关键限速物质, 并且可以通过提供额外的碳源以增强细胞毒性(Lee et al, 2016), 而作为营养盐存在可以保证P. lima维持正常的生长和生理行为。目前由于研究的局限性和基因组信息的缺乏, DSTs的生物合成的机制尚未完全阐明。然而, 毒素的化学结构可以为它们的产生提供一些迹象。OA和DTX1属于聚酮类毒素, 具有这些代谢物的共同结构——环聚醚。研究证明P. lima中含有大量的PKS II(聚酮合酶II)(汤敬谦等, 2009), 荧光标记法(Macpherson et al, 2003)也证明了甘氨酸在DSTs结构中的转化。研究发现P. lima在富含氨基酸的培养基中单细胞产毒总量上升60%~120%甚至对毒素谱产生了影响, 其中在亮氨酸存在的情况下, 最大细胞密度增加约25%, 细胞毒素水平增加一倍(Souto et al, 2001)。综上可见, 氨基酸可能在P. lima生长和毒素生成中发挥重要作用。
2.2 13C-甘氨酸对藻细胞的产毒影响及标记情况分析对gly和13C-gly处理组的OA和DTX1的一级图谱和其[M+NH4]+离子二级图谱进行分析结果见图 3。13C标记对毒素及其酯化成分的保留时间无影响, 批内偏差为0.48%。而13C标记与未标记样品OA和DTX1不同加和方式的离子峰的同位素丰度均发生改变。与未标记样品的相应碎片离子相比, 在质谱图中除了最轻同位素组成的离子所形成的M+0(M)峰外, 还会出现一个或多个重同位素组成的离子峰, 这种离子峰叫做同位素异构体离子峰, 对应的m/z为M+1、M+2、M+3等表示。以[M+NH4]+峰(M)为例, 表现为M+1的响应强度明显上升, M+2、M+3响应强度均有提升, 且出现了可被明显观察到的M+4峰。例如, 13C标记后的OA母离子的精确质量数分别为m/z 823.503 9、m/z 824.506 8、m/z 825.509 9、m/z 826.510 5 ([M+NH4]+)与理论值一致(Δ1.6 mDa: 针对13C1~4- C40~43H68O13+NH4+计算的m/z)。同时在被标记后得二级质谱图中可发现在标记后m/z 267后出现新的268峰, 并且使其脱水峰的同位素分布也发生改变, 13C异构体峰的响应强度显著提升, 且OA出现m/z 753、771和789新的同位素异构体碎片离子。
![]() |
图 3 13C标记前后OA、DTX1的同位素分布及二级质谱图 Fig. 3 The MS/MS spectra of OA and DTX1 and the isotopic distribution by gly and 13C-gly 注: a: gly组OA; b: 13C-gly组OA; c: gly组DTX1; d: 13C-gly组DTX1 |
对标记后化合物去除自然产生的稳定同位素的影响后计算同位素的相对含量(Heuillet et al, 2018)。在2-13C-甘氨酸标记后SHG株产毒量分别为OA (7.31±0.51) ng/cell、DTX1 (4.27±0.21) ng/cell, 2XS株为OA (5.93±0.71) ng/cell。Gly (图 2)与13C-gly组毒素生成量均无显著差异(P<0.05), 表明同位素替代不影响毒素的产生。通过对同位素异构体各质谱峰面积增加值取对数, 可以比较标记后SHG和2XS不同毒素标记后同位素异构体比率的变化(图 4)。Gly组OA和DTX1的lg[M~(M+3)]%=2, 13C-gly标记组DSTs成分M的响应值下降, M+2和M+3显著上升。同时, 酯化态成分也可被2-13C-甘氨酸所标记。其中不同DSTs变化程度有所不同, 上升最高的SHG株OA(图 4b)的3种酯化态成分M+3达到3.30~3.34 [lg(M+3)%]。
![]() |
图 4 13C同位素标记DSTs组分同位素异构体分布情况 Fig. 4 Distribution of isotopic isomers of DSTs components labeled by 13C isotopes 注: a: gly组SHG株; b: 13C-gly组SHG株; c: gly组2XS株; d: 13C-gly组2XS株 |
由于研究的局限性和基因组信息的缺乏, DSTs生物合成的机制尚未阐明(Camacho-Muñoz et al, 2021)。通过用1, 2-13C2-甘氨酸和2-13C, 15N-甘氨酸, 证明DTX-5a和DTX-5b硫化侧链中的氨基酸残基来自甘氨酸(Hu et al, 2017)。诸多学者研究采用如乙酸盐, 乙醇酸等同位素标记试剂实现了DTX5和DTX4的标记(Wright et al, 1996)。如[1-13C]乙酸盐的掺入导致YTX的15个碳的标记(Yamazaki et al, 2011)。在底栖甲藻(Prorocentrum belizeanum)添加[1-13C]乙酸钠和[2-13C]乙酸钠后分别富集了24和38个碳, 证实DTX-5c中68个碳中的62个来自乙酸, 包括所有侧甲基(Vilches et al, 2012)。已经报道了有限数量的此类代谢物的生物合成, 研究在很大程度上受到化合物产量低和同位素标记前体中标记物严重扰乱的阻碍, 因此DSTs的合成机制研究仍处于早期阶段(Kalaitzis et al, 2010)。但相比之下, 本研究中使用2-13C-甘氨酸使DSTs被标记且确定了其标记的碎片离子, 并且首次实现并示踪了多种酯化态的毒素。研究发现二醇酯具有更高的标记比率, 或因其代谢速率或活跃程度所导致(Nagahama et al, 2011; Freund et al, 2017)。Hu等(2017)认为酯化态毒素时P. lima储存的非毒性前体。而Wu等(2020)通过小鼠证明二醇酯类的强毒性。这与通常认为酯化态是储存在叶绿体中非活性前体的结论存在矛盾。此外, 有研究表明P. lima酯化态毒素组成与不同藻株及其细胞生长周期有关(Pan et al, 1999; Wu et al, 2020), 因此借助同位素标记手段进一步研究细胞生长过程中的毒素及同位素丰度变化具有深远的意义。同时应用本方法可获得13C标记的OA和DTX1, 既满足代谢流量分析也可应用于质谱检测内标。13C甘氨酸可以为P. lima的DSTs合成路径的研究提供新方法和思路。
3 结论通过2-13C-甘氨酸培养实现了对利玛原甲藻中的DSTs及其酯化态的同位素标记。甘氨酸显著促进了DSTs及其酯化态成分的合成, 且两种藻株对甘氨酸的响应有所不同。同时鉴定了P. lima酯化态毒素及发现了其标记后同位素丰度的变化, 为后期探究P. lima的产毒通路提供了参考。
古松, 2018. 利玛原甲藻三个ABC转运蛋白全长cDNA序列的获取及其在不同环境条件下的表达分析[D]. 广州: 暨南大学.
|
汤敬谦, 李挺, 杨维东, 等, 2009. 利玛原甲藻中聚酮合酶基因克隆与分析. 生态学报, 29(5): 2383-2390 DOI:10.3321/j.issn:1000-0933.2009.05.024 |
杨维东, 钟娜, 刘洁生, 等, 2008. 不同磷源及浓度对利玛原甲藻生长和产毒的影响研究. 环境科学, 29(10): 2760-2765 DOI:10.3321/j.issn:0250-3301.2008.10.012 |
徐杏, 于仁成, 罗璇, 等, 2012. 温度、盐度和光照对一株有毒利玛原甲藻生长的影响研究. 海洋科学, 36(12): 19-24 |
曾玲, 文菁, 韩冰, 2017. 光照强度对利玛原甲藻生长的影响. 企业科技与发展, (7): 34-37 DOI:10.3969/j.issn.1674-0688.2017.07.010 |
ANTTILA M, STRANGMAN W, YORK R, et al, 2016. Biosynthetic studies of 13-desmethylspirolide C produced by Alexandrium ostenfeldii (= A. peruvianum): rationalization of the biosynthetic pathway following incorporation of 13C-labeled methionine and application of the odd-even rule of methylation. Journal of Natural Products, 79(3): 484-489 DOI:10.1021/acs.jnatprod.5b00869 |
BERG G M, GLIBERT P M, LOMAS M W, et al, 1997. Organic nitrogen uptake and growth by the chrysophyte Aureococcus anophagefferens during a brown tide event. Marine Biology, 129(2): 377-387 DOI:10.1007/s002270050178 |
BRONK D A, 2002. Dynamics of DON [M] // HANSELL D A, CARLSON C A. Biogeochemistry of Marine Dissolved Organic Matter. Amsterdam: Academic Press: 15-249.
|
CAMACHO-MUÑOZ D, PRAPTIWI R A, LAWTON L A, et al, 2021. High value phycotoxins from the dinoflagellate Prorocentrum. Frontiers in Marine Science, 8: 638739 DOI:10.3389/fmars.2021.638739 |
CHO Y, TSUCHIYA S, OMURA T, et al, 2019. Metabolomic study of saxitoxin analogues and biosynthetic intermediates in dinoflagellates using 15N-labelled sodium nitrate as a nitrogen source. Scientific Reports, 9(1): 3460 DOI:10.1038/s41598-019-39708-y |
DAVID H, LAZA-MARTÍNEZ A, KROMKAMP J C, et al, 2018. Physiological response of Prorocentrum lima (Dinophyceae) to varying light intensities. FEMS Microbiology Ecology, 94(1): fix166 |
ECKARDT N A, 2005. Photorespiration revisited. The Plant Cell, 17(8): 2139-2141 DOI:10.1105/tpc.105.035873 |
FLYNN K J, BUTLER I, 1986. Nitrogen sources for the growth of marine microalgae: role of dissolved free amino acids. Marine Ecology Progress Series, 34(3): 281-304 |
FREUND D M, HEGEMAN A D, 2017. Recent advances in stable isotope-enabled mass spectrometry-based plant metabolomics. Current Opinion in Biotechnology, 43: 41-48 DOI:10.1016/j.copbio.2016.08.002 |
GU H F, WU Y R, LÜ S H, et al, 2022. Emerging harmful algal bloom species over the last four decades in China. Harmful Algae, 111: 102059 DOI:10.1016/j.hal.2021.102059 |
GU S, XIAO S W, ZHENG J W, et al, 2019. ABC Transporters in Prorocentrum lima and their expression under different environmental conditions including okadaic acid production. Marine Drugs, 17(5): 259 DOI:10.3390/md17050259 |
HEUILLET M, BELLVERT F, CAHOREAU E, et al, 2018. Methodology for the validation of isotopic analyses by mass spectrometry in stable-isotope labeling experiments. Analytical Chemistry, 90(3): 1852-1860 DOI:10.1021/acs.analchem.7b03886 |
HOU D Y, MAO X T, GU S, et al, 2018. Systems-level analysis of metabolic mechanism following nitrogen limitation in benthic dinoflagellate Prorocentrum lima. Algal Research, 33: 389-398 DOI:10.1016/j.algal.2018.06.004 |
HU T M, LEBLANC P, BURTON I W, et al, 2017. Sulfated diesters of okadaic acid and DTX-1: self-protective precursors of diarrhetic shellfish poisoning (DSP) toxins. Harmful Algae, 63: 85-93 DOI:10.1016/j.hal.2017.01.012 |
KALAITZIS J A, CHAU R, KOHLI G S, et al, 2010. Biosynthesis of toxic naturally-occurring seafood contaminants. Toxicon, 56(2): 244-258 DOI:10.1016/j.toxicon.2009.09.001 |
KILCOYNE J, BURRELL S, NULTY C, et al, 2020. Improved isolation procedures for okadaic acid group toxins from shellfish (Mytilus edulis) and microalgae (Prorocentrum lima). Marine Drugs, 18(12): 647 DOI:10.3390/md18120647 |
LEE T C H, FONG F L Y, HO K C, et al, 2016. The mechanism of diarrhetic shellfish poisoning toxin production in Prorocentrum spp.: physiological and molecular perspectives. Toxins, 8(10): 272 DOI:10.3390/toxins8100272 |
LIM Z F, LUO Z H, LEE L K, et al, 2019. Taxonomy and toxicity of Prorocentrum from Perhentian Islands (Malaysia), with a description of a non-toxigenic species Prorocentrum malayense sp. nov. (Dinophyceae). Harmful Algae, 83: 95-108 DOI:10.1016/j.hal.2019.01.007 |
MACPHERSON G R, BURTON I W, LEBLANC P, et al, 2003. Studies of the biosynthesis of DTX-5a and DTX-5b by the dinoflagellate Prorocentrum maculosum: regiospecificity of the putative Baeyer-Villigerase and insertion of a single amino acid in a polyketide chain. The Journal of Organic Chemistry, 68(5): 1659-1664 DOI:10.1021/jo0204754 |
MOREIRA-GONZÁLEZ A R, FERNANDES L F, UCHIDA H, et al, 2019. Variations in morphology, growth, and toxicity among strains of the Prorocentrum lima species complex isolated from Cuba and Brazil. Journal of Applied Phycology, 31(1): 519-532 DOI:10.1007/s10811-018-1587-y |
MOREIRA-GONZÁLEZ A R, ROSA K M S, MAFRA L L, 2022. Prevalence of okadaic acid in benthic organisms associated Prorocentrum lima complex in a sub-tropical estuary. Food Additives & Contaminants: Part A, 39(2): 382-396 |
MULHOLLAND M R, BONEILLO G, MINOR E C, 2004. A comparison of N and C uptake during brown tide (Aureococcus anophagefferens) blooms from two coastal bays on the east coast of the USA. Harmful Algae, 3(4): 361-376 DOI:10.1016/j.hal.2004.06.007 |
NAGAHAMA Y, MURRAY S, TOMARU A, et al, 2011. Species boundaries in the toxic dinoflagellate Prorocentrum lima (Dinophyceae, Prorocentrales), based on morphological and phylogenetic characters. Journal of Phycology, 47(1): 178-189 DOI:10.1111/j.1529-8817.2010.00939.x |
NISHIMURA T, UCHIDA H, NOGUCHI R, et al, 2020. Abundance of the benthic dinoflagellate Prorocentrum and the diversity, distribution, and diarrhetic shellfish toxin production of Prorocentrum lima complex and P. caipirignum in Japan. Harmful Algae, 96: 101687 DOI:10.1016/j.hal.2019.101687 |
PAN Y, CEMBELLA A D, QUILLIAM M A, 1999. Cell cycle and toxin production in the benthic dinoflagellate Prorocentrum lima. Marine Biology, 134(3): 541-549 DOI:10.1007/s002270050569 |
SOUTO M L, FERNÁNDEZ J J, NORTE M, et al, 2001. Influence of amino acids on okadaic acid production. Toxicon, 39(5): 659-664 DOI:10.1016/S0041-0101(00)00190-2 |
SUN Q S, FAN T W M, LANE A N, et al, 2020. Applications of chromatography-ultra high-resolution MS for stable isotope-resolved metabolomics (SIRM) reconstruction of metabolic networks. TrAC Trends in Analytical Chemistry, 123: 115676 DOI:10.1016/j.trac.2019.115676 |
TAYLOR G T, GOBLER C J, SAÑUDO-WILHELMY S A, 2006. Speciation and concentrations of dissolved nitrogen as determinants of brown tide Aureococcus anophagefferens bloom initiation. Marine Ecology Progress Series, 312: 67-83 DOI:10.3354/meps312067 |
VILCHES T S, NORTE M, DARANAS A H, et al, 2012. Biosynthetic studies on water-soluble derivative 5c (DTX5c). Marine Drugs, 10(10): 2234-2245 |
WRIGHT J L C, HU T, MCLACHLAN J L, et al, 1996. Biosynthesis of DTX-4: confirmation of a polyketide pathway, proof of a baeyer−villiger oxidation step, and evidence for an unusual carbon deletion process. Journal of the American Chemical Society, 118(36): 8757-8758 DOI:10.1021/ja961715y |
WU H Y, CHEN J Q, PENG J X, et al, 2020. Nontarget screening and toxicity evaluation of diol esters of okadaic acid and dinophysistoxins reveal intraspecies difference of Prorocentrum lima. Environmental Science & Technology, 54(19): 12366-12375 |
YAMAZAKI M, IZUMIKAWA M, TACHIBANA K, et al, 2012. Origins of oxygen atoms in a marine ladder-frame polyether: evidence of monooxygenation by 18O-labeling and using tandem mass spectrometry. The Journal of Organic Chemistry, 77(11): 4902-4906 DOI:10.1021/jo300531t |
YAMAZAKI M, TACHIBANA K, SATAKE M, 2011. Complete 13C-labeling pattern of yessotoxin a marine ladder-frame polyether. Tetrahedron, 67(5): 877-880 DOI:10.1016/j.tet.2010.12.015 |
YAO X, ZHU G W, CAI L L, et al, 2012. Geochemical characteristics of amino acids in sediments of Lake Taihu, a large, shallow, eutrophic Freshwater Lake of China. Aquatic Geochemistry, 18(3): 263-280 DOI:10.1007/s10498-012-9160-9 |
ZHANG H, LI Y, CEN J Y, et al, 2015. Morphotypes of Prorocentrum lima (Dinophyceae) from Hainan Island, South China Sea: morphological and molecular characterization. Phycologia, 54(5): 503-516 DOI:10.2216/15-8.1 |